Laser
What is a Laser?
The word “laser” is an acronym for Light Amplification by Stimulated Emission of Radiation. All lasers convert input energy into light through the process of stimulated emission.
Lasers range in size from small semiconductor devices to huge systems that fill an entire building. They also utilize diverse gain materials – from free electrons to solids. But all these different lasers operate on the same underlying principles.
The phenomenon of “stimulated emission” is at the heart of laser operation. To create the conditions that produce and maintain stimulated emission, lasers incorporate three key functional elements. These are:
- A gain medium which has the ability to support a population inversion
- A pump source which supplies the energy to create the population inversion
- A resonant cavity which provides a feedback mechanism to support amplification, and also determines the spatial and spectral properties of the laser beam
However, the form and implementation of these three elements differ greatly from one type of laser to another. Specifically, this includes the use of different types of laser materials (gain media that support stimulated emission), how the energy is supplied to this material, forms of the laser cavity, and the output characteristics.
Let’s look at the underlying principles of each of these elements, and some of the forms they take in various types of lasers.
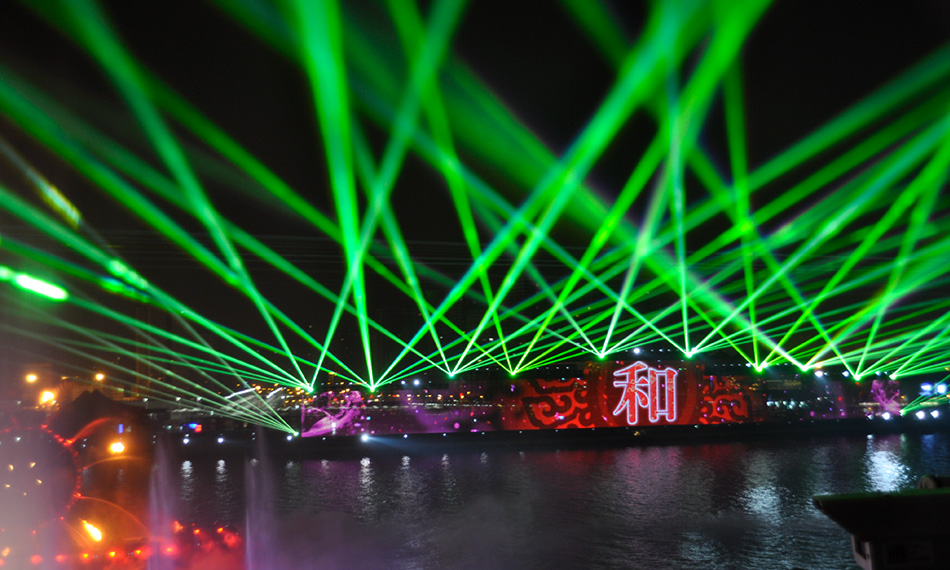
Courtesy: LaserAnimation Sollinger GmbH
Laser Gain Media
To understand what a laser gain material is, it’s first necessary to understand the process of stimulated emission. Quantum mechanics tells us that atoms and molecules can only exist at certain specific, discrete energy levels. The lowest energy level is called the ground state, while higher energy levels are known as excited states.
Normally, the temperature of a material determines how its atoms or molecules are distributed among the possible energy levels. In a typical thermal equilibrium situation, most atoms or molecules are in the lower energy states, and progressively fewer are in the excited states.
In some materials, it is possible to supply energy (a process called “pumping”) to create a population inversion. This means that more than 50% of the atoms or molecules are in the excited state – the opposite of the normal thermal equilibrium situation.
A population inversion creates favorable circumstances for the process of stimulated emission. The process starts when one atom or molecule emits a photon and drops from a higher to a lower energy state. This is called spontaneous emission.
This first photon passes near another atom or molecule and stimulates it to emit a second photon. The second photon has identical energy, direction, phase, and polarization as the stimulating photon. These two photons then cause stimulated emission of two more photons – so now there are four photons. This process rapidly cascades to create a large number of identical photons. This cascade of photons – called amplification or gain – is the basis of laser action. It allows pump energy to be converted into coherent laser light.
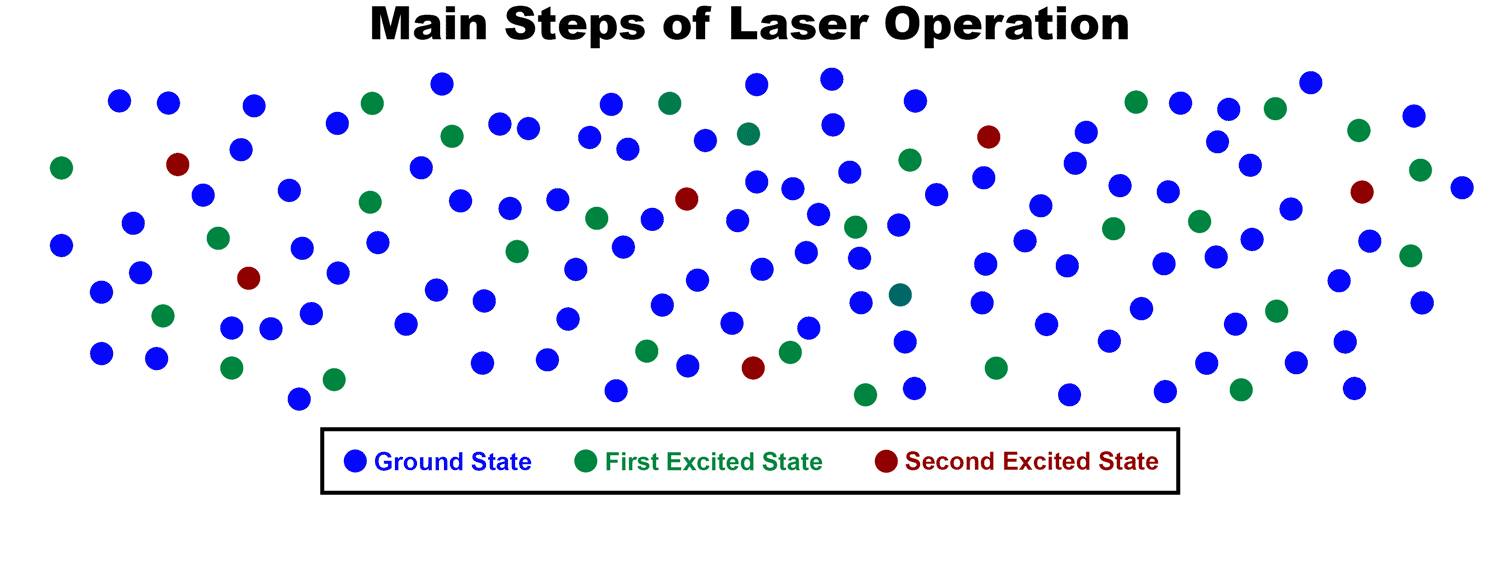
However, not all materials can support a population inversion and stimulated emission. The capability to do this depends on several factors, including the allowable energy levels in the atoms or molecules, the transition probabilities between these energy levels, the lifetime of excited states (how long the atom or molecule tends to remain in that excited state), and several other factors.
Materials that can support gain come in virtually every form of matter – solid, liquid, and gas. By convention, these are typically grouped into categories as shown in the table.
Gain Medium |
Typical Examples |
Gas |
Carbon Dioxide (CO2), Excimer, Argon-Ion, Helium-Neon (HeNe) |
Liquid |
Fluorescent dye |
YAG (Nd:YAG), Vanadate (Nd:YVO4), Titanium:Sapphire (Ti:S), Yb:glass |
|
Er-doped fiber, Yb-glass fiber |
|
Semiconductor Diode |
Diode Laser, Optically Pumped Semiconductor Laser (OPSL), Vertical Cavity Surface-Emitting Laser (VCSEL) |
Free-electrons |
Free-electron Laser (FEL) |
Laser Pumping
Energy must be supplied from an external source into the gain medium to produce the population inversion. (This process leads to stimulated emission, which produces laser output). The method depends on the gain medium. Most commonly, the energy is supplied in the form of electricity or light. A less common method is the energy released from an exothermic chemical reaction.
All the various solid-state crystal and optical fiber gain media are electrical insulators; in other words, they can’t conduct an electric current. So, these laser materials must be pumped optically. That is, an external light source is focused into the gain medium, and the atoms or molecules of the laser material absorb this light. The result: the atoms or molecules reach the necessary excited state.
The earliest solid-state lasers used flashlamps as a pump source, and these are still in use for some applications. Their main advantage is low cost and the ability to deliver high laser-pulse energy.
But flashlamps produce a broad spectrum of light. The laser gain material can only utilize a very narrow spectrum of this light – specifically, the wavelength that corresponds to the energy difference between the ground state and highest excited state. In fact, most of the flashlamp pump energy is wasted, and this makes these lasers electrically inefficient, which means they produce a lot of waste heat. As a result, fairly substantial cooling system is needed to eliminate this heat.
It is now more common to pump solid-state and fiber lasers with yet another laser – typically, a diode or solid-state laser. The wavelength of the pump laser is specifically chosen to match the absorption of the gain medium. This produces much higher overall pumping efficiency and reduces the cooling requirements.
There’s an additional advantage to the use of a laser as a pump source. Most lasers produce an easily focused beam. This allows the pump light to be concentrated in the gain medium right where it does the most good. That is, within what is called the “mode volume.” This is the region within the gain medium actually occupied by the laser beam. Pump light that goes into other parts of the laser medium is wasted. Effectively filling the mode volume maximizes laser efficiency, and also improves the output beam quality.
Fiber lasers that use fiber-coupled diode lasers as the pump source are a good example of this principle. These can easily be configured so the pump light is directed primarily into the core or cladding of the gain fiber, as needed — and the result is a highly efficient laser system.
Electrical pumping can be used with semiconductor (diode) lasers, since these are devices specifically intended to conduct electricity. In particular, they consist of a semiconductor p-n junction which is forward-biased. The applied voltage supplies energy to promote enough electrons from the semiconductor’s valence band into its conduction band to create a population inversion. Photons are emitted when the electrons and holes (absence of an electron in the valence band) recombine, and the population inversion enables stimulated emission to occur.
It's also possible to optically pump a semiconductor laser. In this case, the output of another diode laser is focused into the active region of the diode. This supplies the pump energy, instead of using electrical current. Optical pumping makes the semiconductor laser more complex but can deliver a wider choice of laser output wavelengths, higher output power, and better efficiency (meaning less heat generation).
Electrical pumping of gas lasers is somewhat more complex. Gas lasers usually consist of several gases contained within a laser tube. A high voltage is used to create an electron discharge within the laser tube. These high-energy electrons impact the gas molecules and impart energy to them.
In the case of CO2 lasers, the electrons collide with nitrogen molecules and vibrationally excite them. These nitrogen molecules subsequently collide with CO2 molecules, transferring energy to the CO2 molecules to create a population inversion.
Another example is the ion laser. In these, an electron discharge again creates collisions with the Argon or Krypton gas within the laser tube. The first collision ionizes the gas. Then, further collisions with the ions provide energy to put them in an excited state and produce a population inversion.
Laser Type |
Typical Pump Source |
Electrical discharge |
|
Electrical current (diode laser), Diode laser (OPSL, VCSEL) |
|
Electrical discharge |
|
Diode laser |
|
Electrical discharge |
|
Flashlamp, Diode laser |
|
Solid-state (Nd:YVO4) laser, OPSL |
Resonant Cavities
Typically, a resonant cavity (or resonator) is used to make photons pass through the gain medium numerous times before exiting the laser. This is necessary to build up a useful level of laser output, because the amount of amplification on each pass through the gain medium is relatively small. One major exception to this is the excimer laser, which imparts a very large amount of gain (amplification) in even a small number of passes.
The simplest type of resonant cavity is built with two mirrors facing each other, and the laser gain medium placed in between them. The rear mirror reflects as near to 100% as possible. The front mirror – called the output coupler – might have a reflectance of anywhere from 30% to 99% depending on the gain medium.
In operation, light bounces back and forth between these mirrors, gaining intensity with each pass through the laser medium. Some of the light exits the resonator through the output coupler. As a result, the light intensity within the laser resonator is always much higher than the intensity of light that comes out of the device.
The end mirrors often have a curvature on them to spatially confine the light (to keep photons from “walking out” of the resonator after multiple passes) and to define the shape of the beam.
Laser Resonator Basics
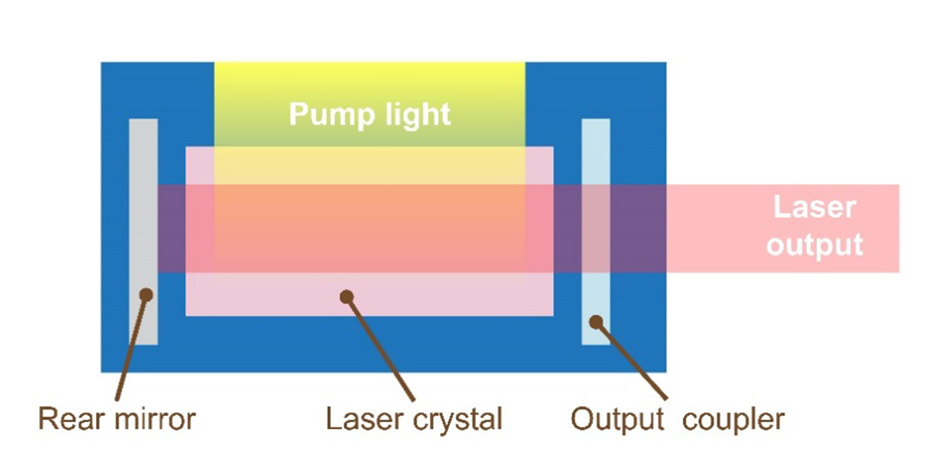
The main elements of a laser resonator. A pump source supplies energy into a gain medium which is placed between mirrors. The mirrors provide feedback, making emitted photons pass multiple times through the gain material for amplification.
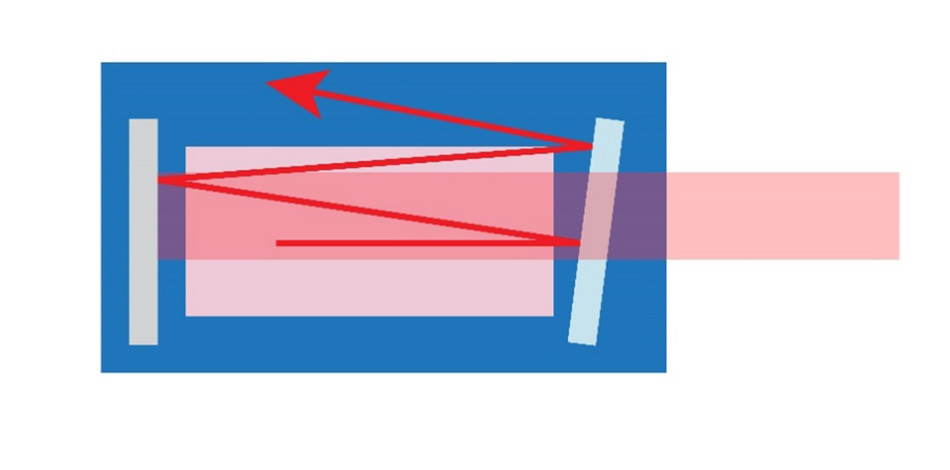
A resonator using two flat mirrors is simple to construct, but is very sensitive to misalignment since it causes a photon to "walk-off" after a small number of passes. However, if the resonator is physically small, this isn't a problem. This configuration is commonly used in diode lasers.
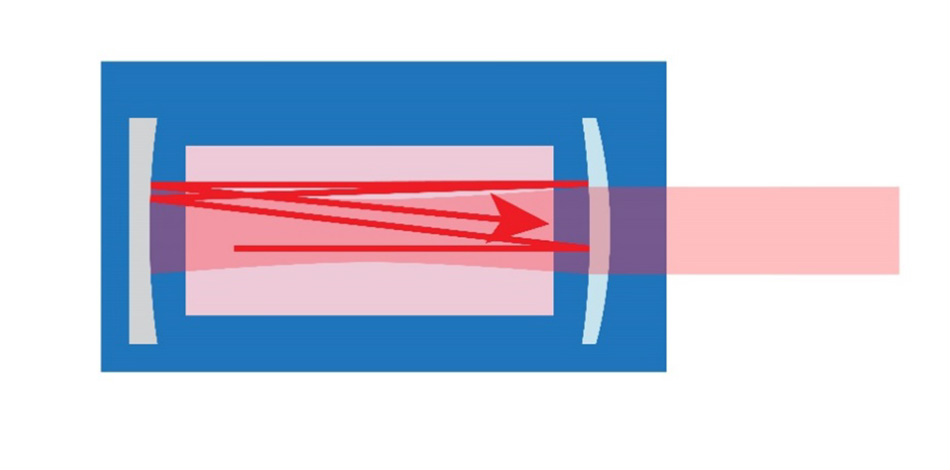
Making one or both of the mirrors concave better confines the beam to within the resonator, and also yields a laser with a small, well-formed beam. Variations on this resonator design are common for many solid-state and gas lasers.
In a fiber laser, the mirrors are often high-reflectance Fiber Bragg Gratings (FGBs) integrated directly into the fiber. In this case, the fiber itself spatially confines the beam and defines its shape. In diode lasers, the mirrors are formed by cleaving the ends of the semiconductor device and applying optical thin film coatings to them.
The Unique Characteristics of Laser Light
Lasers have become indispensable tools in a diverse range of applications. The truth is their operating principles and construction offer a unique set of beam characteristics that cannot be duplicated by any other technology. Some of the most important properties are described here.
Property |
Explanation |
Applications |
Coherence |
Stimulated emission produces photons that are all in phase with each other. This is called “coherence.” This property enables laser light to produce well-defined interference fringe patterns. |
|
Directionality |
The mechanism of stimulated emission and the characteristics of most laser resonators frequently combine to yield a highly directional beam that does not spread quickly over distance. |
|
High intensity |
Laser light can be highly intense, because all the laser power can readily be concentrated (focused) into a small spot. |
|
Monochromaticity |
Stimulated emission produces photons that all have the same single wavelength or a very narrow range of wavelengths. |
|
The first laser was demonstrated in 1960. While it generated some interest and excitement, it largely remained a “solution in search of a problem” for the first several years. But, bit-by-bit, practical applications for laser were developed. Today, lasers are common and found in an extraordinarily diverse range of applications.
Lasers count your blood cells when you have lab tests. Lasers are used to project movies at many theaters. Lasers are used to perform countless surgeries and other medical procedures every year. Lasers weld automobiles and are key in the production of electric vehicles. Lasers carry virtually every phone call and all internet traffic over fiber optic cables. Lasers make the microelectronic circuits that power all modern technology. Many people carry lasers with them all the time – since they’re incorporated into some cell phones for distance measurement. Lasers mark many of the packages used for consumer goods with information like date codes and serial numbers. Lasers have numerous uses in scientific research, from cutting-edge neuroscience, microscopy, and spectroscopy, to gravity wave astronomy. Today, lasers really do light the way towards a brighter future.